Exploring the Mechanics of Nuclear Propulsion Systems
Written on
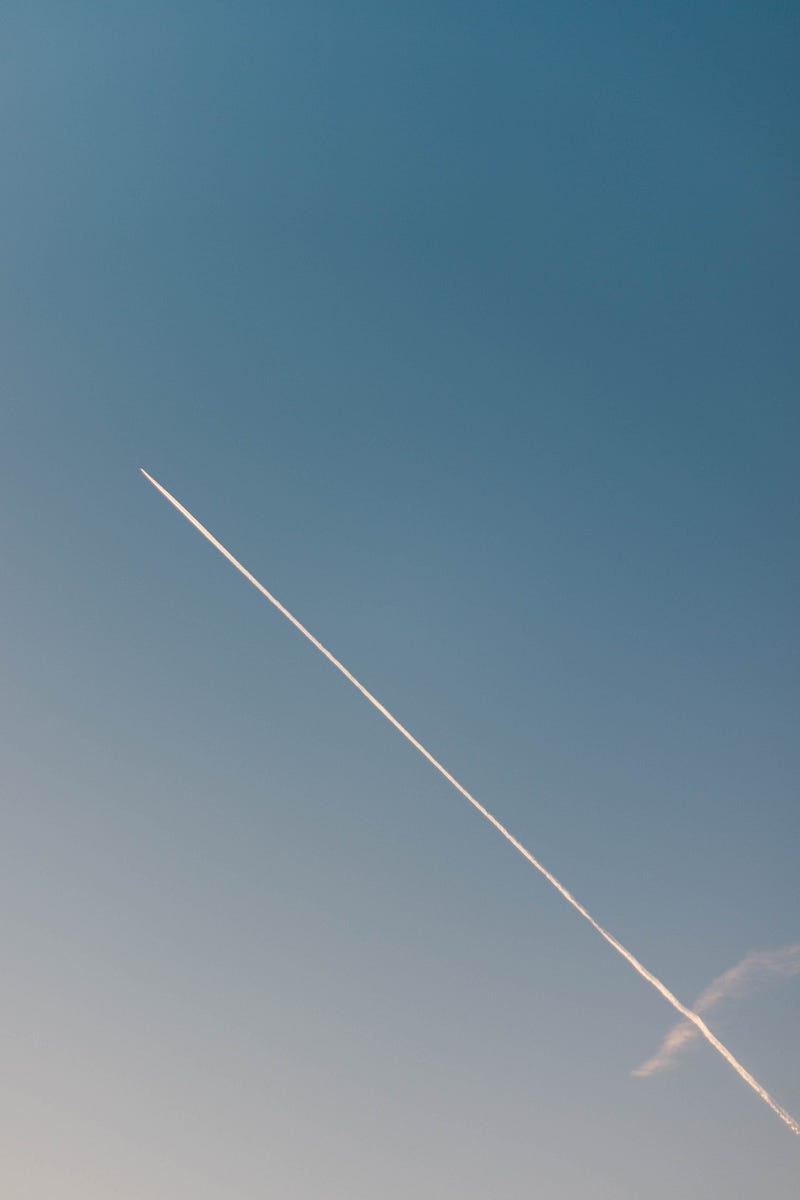
In recent news, you may have encountered reports regarding a nuclear incident at Nyonoksa, Russia, linked to a malfunction during a test of a nuclear-powered engine thought to be associated with the 9M730 Burevestnik cruise missile. This missile is said to feature an unlimited range while airborne, owing to its nuclear propulsion system. So, how do these engines function, and what is their historical context?
History
The concept of harnessing nuclear energy for vehicle propulsion is not a contemporary notion; various countries have explored this idea over the decades. Examples include:
- Nuclear-powered automobiles
- Nuclear-powered aircraft
- Nuclear-powered ships
- Nuclear-powered spacecraft
- Nuclear-powered missiles
However, numerous challenges have hindered the widespread adoption of these technologies, including concerns about nuclear proliferation, strategies for accident management (such as preventing reactor leaks in the event of a crash), and issues related to shielding and weight. Manned vehicles often face significant weight constraints since adequate shielding to protect crew members can add considerable mass, making flight impractical.
In contrast, maritime vessels can better accommodate weight due to their larger capacities, and applications without crew (like missiles and spacecraft) typically do not require shielding once airborne (though launch facilities may necessitate protective measures for personnel). Indeed, spacecraft and rovers frequently utilize radioisotope thermoelectric generators (RTGs) in scenarios where batteries or solar panels fall short. RTGs generate power by converting heat from radioactive decay, helping to maintain electronic functionality (e.g., the Curiosity Rover). Additionally, RTGs are employed on Earth, such as in automated lighthouses in Soviet regions.
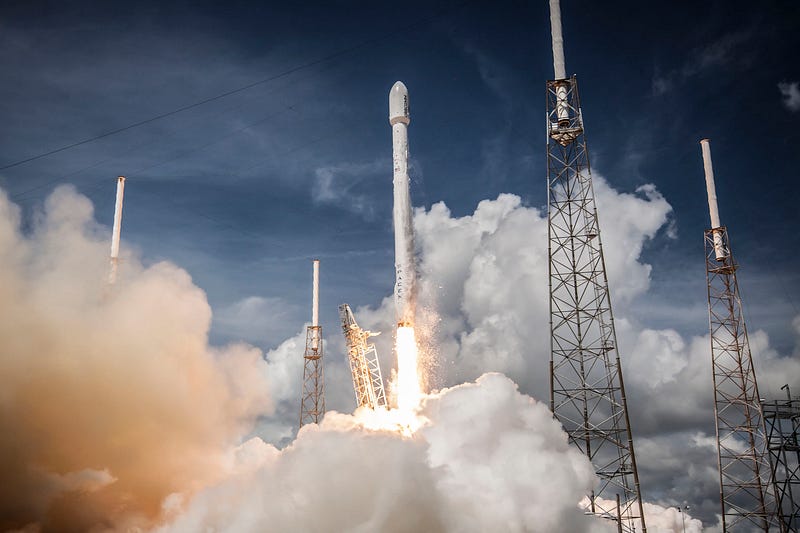
How Does a Nuclear Reactor Provide Unlimited Range to Missiles or Aircraft?
To grasp this, we must examine the fundamentals of rocket propulsion for space exploration. At its core, the engine comprises a chamber where fuel and oxidizer combust, generating hot gases that expand and escape through a strategically designed opening. The ejected gases propel the engine (and its craft) in the opposite direction.
This phenomenon aligns with Newton’s Third Law of Motion, which asserts:
> "For every action, there is an equal and opposite reaction."
This principle is often demonstrated in classrooms, where a teacher in a wheeled chair uses a fire extinguisher to propel themselves across the room. The gas expelled from the extinguisher applies force, pushing the teacher away. The key takeaway is that increasing the volume of gas released, the mass of the expelled material, or the speed of ejection enhances the resulting force.
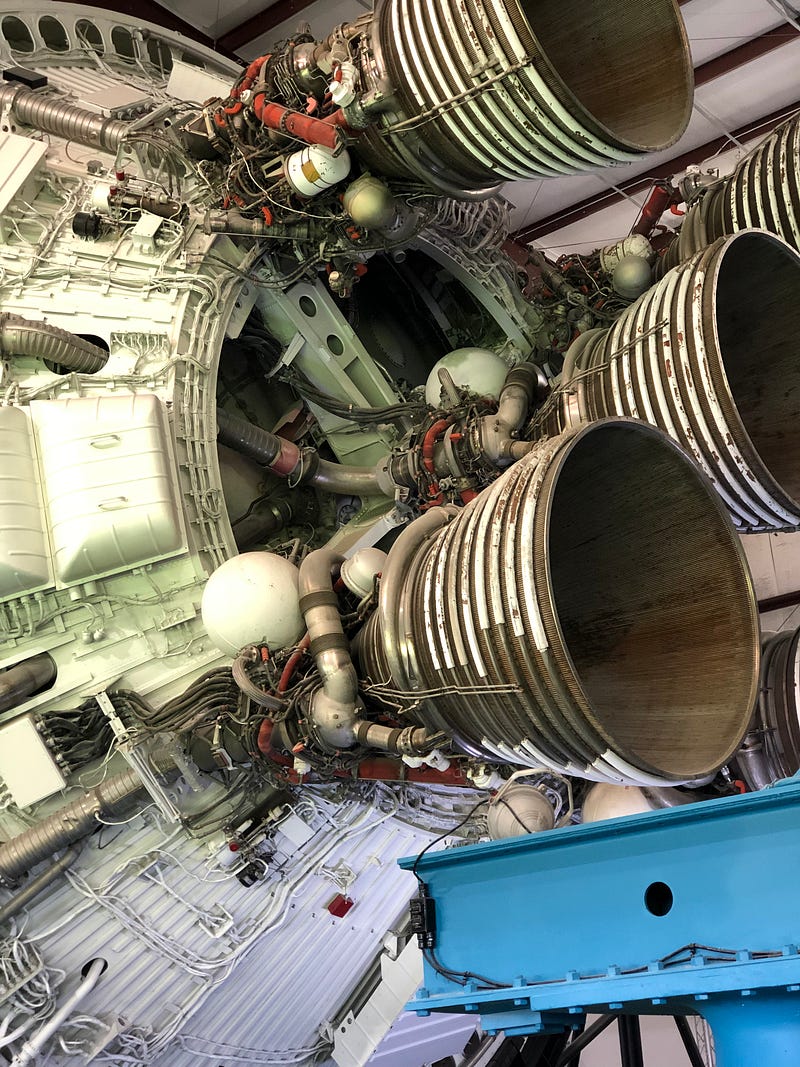
How Can You Enhance Rocket Exhaust Velocity?
The speed at which exhaust gases exit the engine is influenced by the energy imparted by the heat generated during combustion. Burning more fuel results in increased energy for the gases, but it also produces a greater volume of exhaust, thus raising the overall mass expelled.
Another approach is to enhance the fuel's combustion efficiency, allowing it to burn at significantly higher temperatures or contain more energy. However, high-energy rocket fuels often come with increased costs and safety risks (e.g., corrosive properties or extreme temperatures like liquid oxygen).
In the realm of space exploration, these challenges are often considered acceptable, as optimizing fuel and engine design to maximize performance is crucial. If a fuel can provide the same thrust while weighing half as much as a previous version, the rocket requires less fuel, reduces overall weight, and is more economical for launches. This also translates to extended operational life for spacecraft, as satellites are frequently decommissioned due to fuel depletion rather than mechanical failures.
How Does This Apply to Aircraft?
The principles governing space travel are similarly applicable to aircraft operating within the atmosphere. The less fuel required for a specific distance (through either engine or fuel advancements), the farther an aircraft can fly before needing to refuel.
Aircraft also benefit from the ability to draw in air to mix with fuel, negating the necessity to carry oxidizer as spacecraft must do in the vacuum of space. This further enhances their range compared to systems that must transport both fuel and oxidizer.
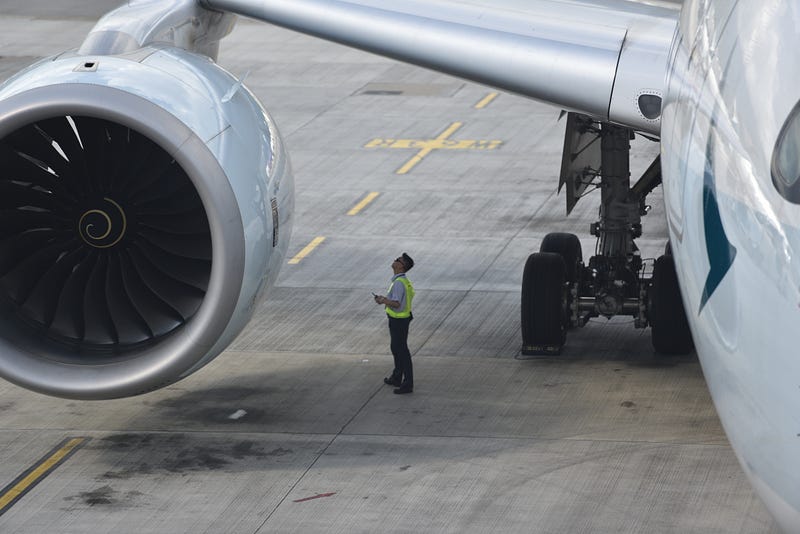
Why Do Missiles Utilize Rocket Engines?
Rockets often feature simpler designs compared to the intricate mechanisms found in commercial aircraft engines, making them easier to store and rapidly deploy.
Conventional aircraft engines require complex fan assemblies (as seen in commercial jet engines) and ductwork to compress incoming air, ensuring efficient combustion with fuel. These engines also face speed limitations before mechanical components fail or heat causes material degradation.
As previously mentioned, increasing fuel combustion temperature yields more energy for exhaust gases. However, such engines must be designed to withstand the stresses of operating at elevated temperatures.
How Do Nuclear Engines Function?
In a nuclear reactor, fuel undergoes fission when struck by neutrons in a controlled environment, releasing energy in amounts far surpassing that of even the most advanced rocket fuels.
During the Cold War, three primary designs for nuclear rocket engines were developed: solid-core, liquid-core, and gas-core engines. While each operates uniquely, they share the fundamental principle of introducing a propellant into the reactor to absorb heat and then expel it at high velocity, propelling the engine forward.
Solid-Core Nuclear Rocket
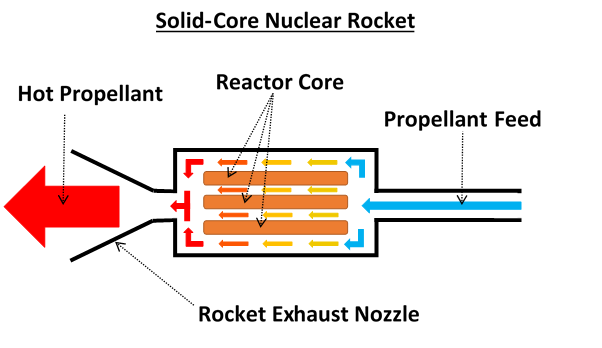
This is the most conventional and straightforward design. In this configuration, a nuclear reactor core heats the propellant, which is then expelled through the rocket nozzle. The fuel remains solid, ensuring the propellant does not come into direct contact with it, and the exhaust is free of radioactive materials. This feature is crucial for terrestrial applications, as radioactive fallout from a nuclear rocket would be undesirable.
The primary limitation of this design is the requirement for the fuel to remain solid, which restricts the reactor's maximum temperature before melting occurs. This, in turn, limits the energy output and the potential speed of the propellant.
Liquid-Core Nuclear Rocket
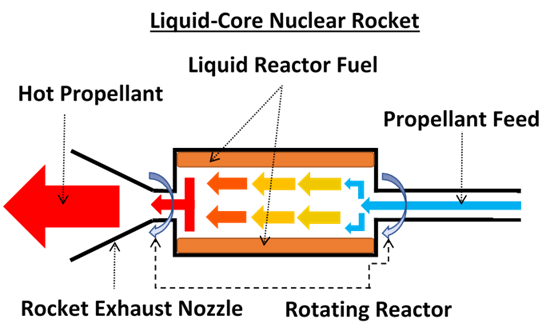
In this design, the nuclear fuel can reach much higher temperatures as it can become liquid. The reactor spins at high speeds to keep the fuel against the reactor walls, preventing loss and ensuring efficiency. It is vital that no radioactive materials escape, which adds to the complexity of the design.
Gas-Core Nuclear Rocket
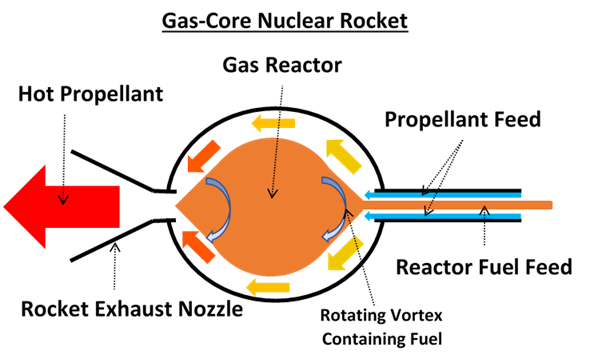
This approach elevates temperatures even further by allowing the fuel to vaporize. To contain the gaseous fuel, a vortex is required, which complicates the design and necessitates careful management of both nuclear fuel and propellant to prevent mixing and loss.
Nuclear Engine Applications on Earth
As explored, the three fundamental designs exist, but the solid-core reactor is likely to be the most feasible for practical use. Its simplicity and safety make it an attractive option, minimizing the risk of radioactive emissions.
This design allows for easier transportation and containment, with heat transferred through a heat exchange system, further reducing the chance of nuclear material escaping.
While the reactor poses substantial risks in the event of a crash and is more costly than traditional engines, these concerns may be overshadowed by the potential consequences of a nuclear explosion, especially if utilized to carry a nuclear warhead.
The designs discussed thus far typically use onboard propellant, limiting range based on available supply. However, adapting the system to utilize ambient air could extend range, contingent on the reactor's operational lifespan.
The most likely adaptation would be to a ramjet design, potentially similar to that used in the 9M730 Burevestnik cruise missile, which may have been linked to the recent nuclear engine incident.
Nuclear Ramjets
In a conventional jet engine, incoming air must be compressed prior to mixing with fuel for combustion, introducing complexity.
A ramjet simplifies this process by using the craft's forward motion to compress air, making the engine lighter and easier to integrate into missile designs, though it necessitates reaching a specific speed to ensure adequate air compression. To achieve this speed, a booster rocket engine is often employed, which can be discarded once the desired velocity is attained (similar to a JATO system on aircraft).
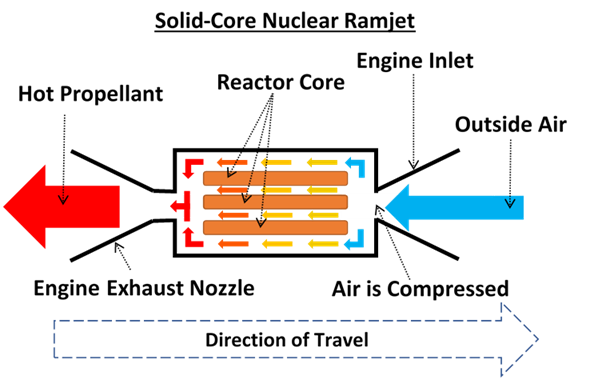
Summary
In summary, we have covered:
- A brief history of nuclear propulsion
- The application of nuclear reactors for travel
- Basic designs of nuclear engines
- Insights into the most promising design for terrestrial use
I hope you found this article engaging, as I enjoyed crafting it. Until next time!