Understanding the Expansion of the Universe: A New Era in Astronomy
Written on
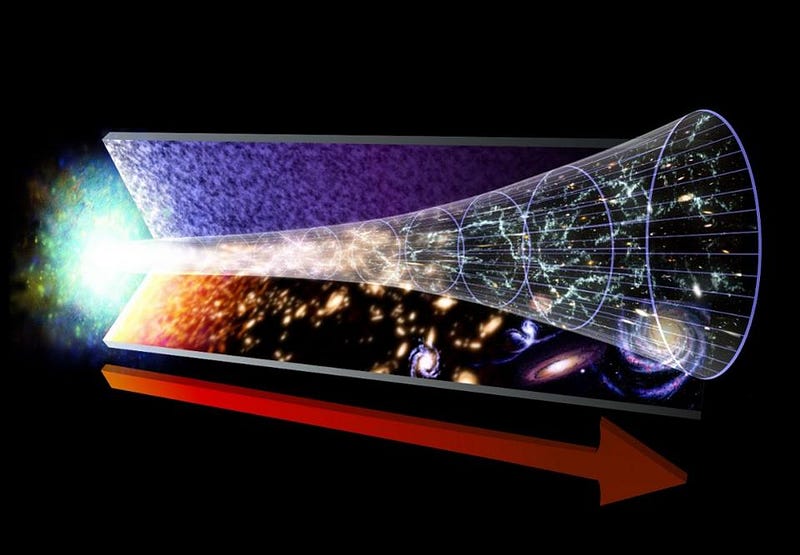
# Understanding the Expansion of the Universe: A New Era in Astronomy
And if the data is good enough, we can determine that it’s accelerating directly, too, silencing the last remaining doubters.
To grasp the essence of the Universe's composition, its destiny, or the timeline of the Big Bang, two fundamental pieces of information are essential. According to physical cosmology, these are:
- The current rate of the Universe's expansion.
- The variation in this expansion rate over time.
This knowledge enables us to reconstruct the Universe’s makeup, its past, and its future trajectory.
Historically, significant debate has surrounded these matters, as various teams employing different methodologies yield divergent results. Yet, they all share a commonality: their measurements have relied solely on indirect techniques to ascertain the Universe's expansion over time. However, with a new generation of telescopes becoming operational in the 2020s, astronomers will finally have the tools to gauge the expansion rate directly. The science behind this advancement is remarkable.
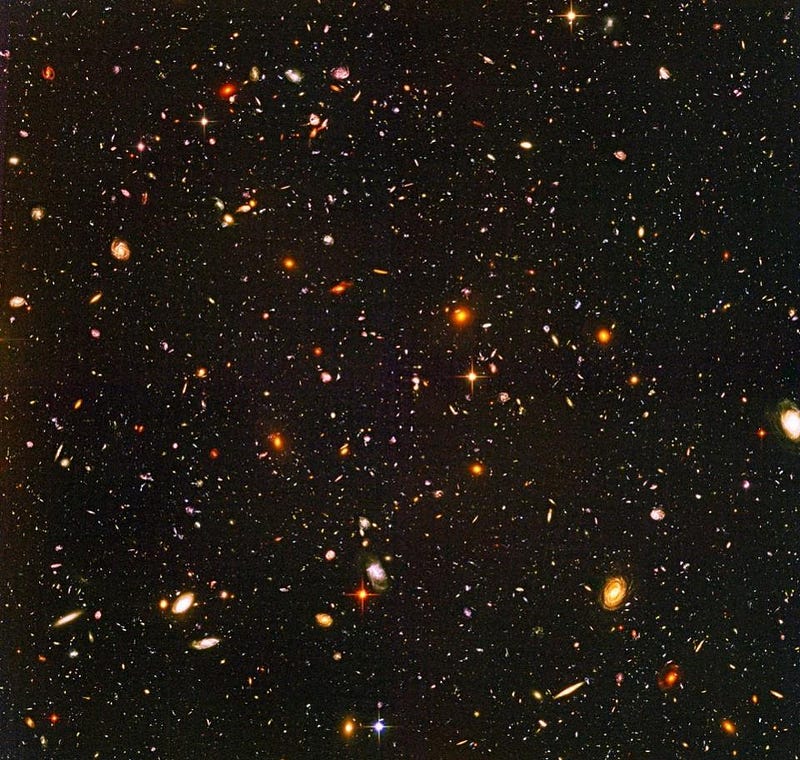
In an expanding Universe, light from a distant galaxy will appear altered to an observer far away. At any given moment, the emitted light from stars and galaxies has certain characteristics. Specifically, it behaves as if it consists of multiple blackbody emissions—similar to how perfectly dark objects emit radiation when heated—layered over each other.
If this was the sole light the Universe provided, it would be exceedingly difficult to measure its expansion. Even if we devised ingenious methods to assess distances to these remote objects, accurately gauging the effects of an expanding Universe would still pose challenges. As the Universe expands, light stretches while traveling from its source to the observer; without understanding the intrinsic properties of that light, we couldn't reliably measure the extent of its stretching.
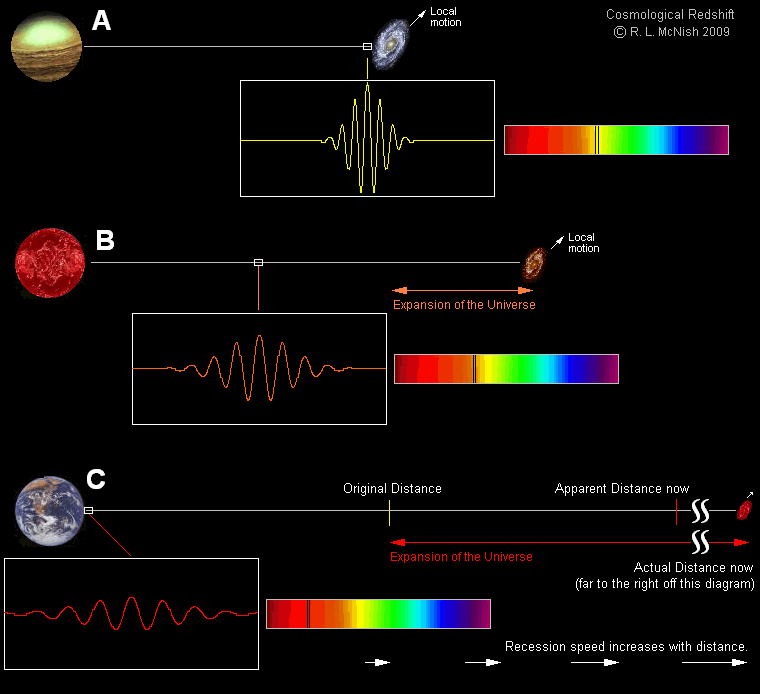
Fortunately, our Universe is not solely composed of stars and galaxies radiating at specific temperatures; it also contains atoms. Atoms uniquely absorb or emit radiation at particular wavelengths that correspond to their atomic and molecular transitions.
By analyzing light from various sources—ranging from our Sun to the most distant galaxies and quasars—we can detect the absorption and emission lines caused by the atoms present in these objects. Two factors contribute to the extent of redshift: the motion of the light source relative to the observer and the expansion of space during the light's journey.
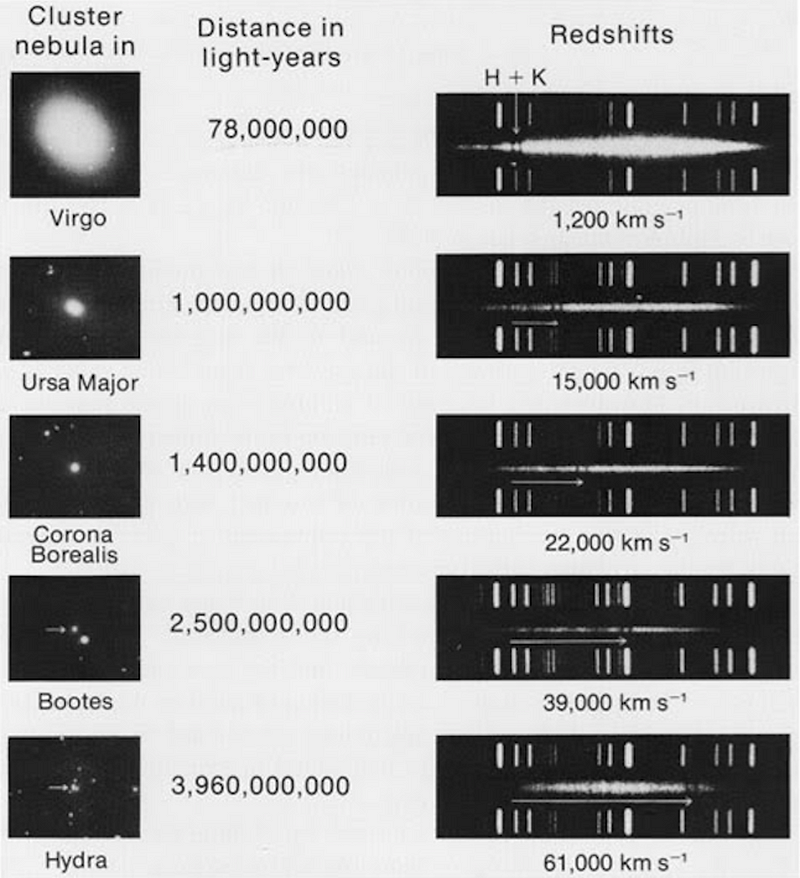
First identified by Vesto Slipher in 1917, some observed objects display spectral signatures of particular atoms, ions, or molecules, systematically shifted towards either the red or blue ends of the spectrum. This data, when combined with Hubble's distance measurements, laid the foundation for the concept of an expanding Universe: the greater the distance of a galaxy, the more pronounced its redshift.
By integrating distance and redshift measurements, we can reconstruct the Universe's expansion, employing various methods to ascertain distances to a range of celestial objects. When we amalgamate data from all observable objects with reliable distance and redshift measurements, we derive stringent constraints on the Universe's expansion history. Since matter and radiation dilute in specific ways as the Universe expands, and dark energy maintains a constant energy density, this information collectively informs us about the Universe's composition, its current expansion rate, and its evolution over time.
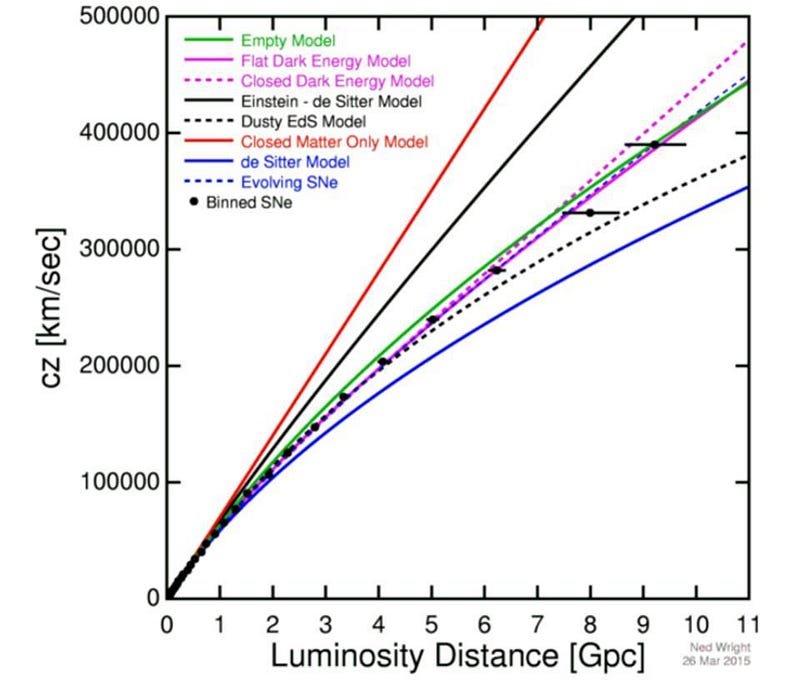
This achievement represents a significant milestone in cosmology, providing answers to longstanding questions with unprecedented precision, albeit with some uncertainties and controversies. However, confidence in these indirect measurements is inherently limited. In astronomy, the vast distances and scales involved often preclude real-time observations of changes.
If we envision the fabric of space as a dough ball, with galaxies as raisins, then the expanding Universe resembles dough leavening. The raisins (galaxies) appear to drift apart, with more distant ones receding more swiftly. This observation largely stems from the expanding dough (space) rather than the movement of the raisins (galaxies), which remain stationary within their local regions.
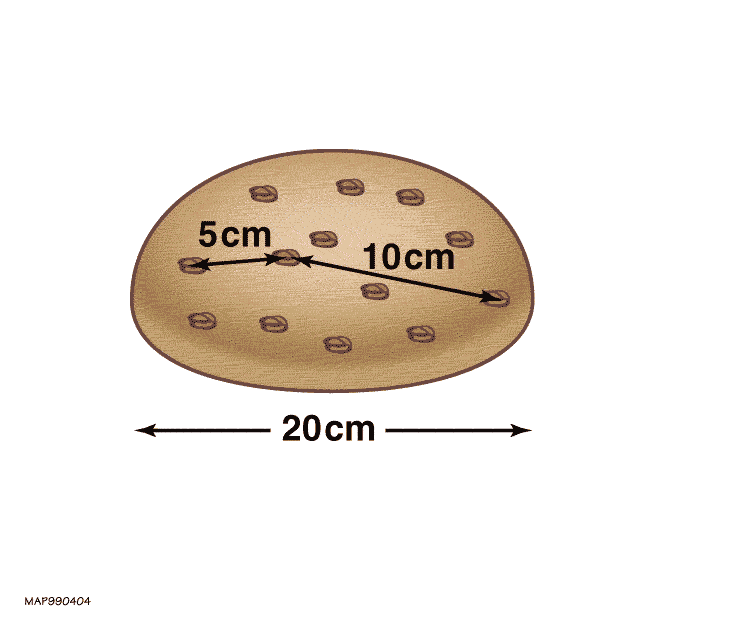
This analogy explains why measuring the redshifts and distances of numerous objects enables us to reconstruct the Universe's expansion throughout its history. The convergence of various data sets supporting an expanding, uniformly filled Universe within the framework of relativity bolsters confidence in our cosmological model.
However, just as the scientific community awaited direct measurements of gravitational waves by LIGO, there remains the potential for errors in our interpretations of the Universe's properties. If we could observe a distant object, record its redshift and distance, and later return to assess any changes, we would have the opportunity to directly measure the Universe's expansion for the first time.
Given that our leading model posits the Universe is approximately 13.8 billion years old, measuring significant expansion over human timescales poses challenges. For the most distant galaxies and quasars—tens of billions of light-years away—the expected change in redshift over time equates to just 1 cm/s per year.
Even with the most advanced telescopes today, we can only resolve redshifts to about 100 to 200 cm/s, necessitating centuries of observation to detect changes in distant objects. While numerous distant objects have been identified, our technological capabilities currently fall short of achieving the precision required for such measurements.
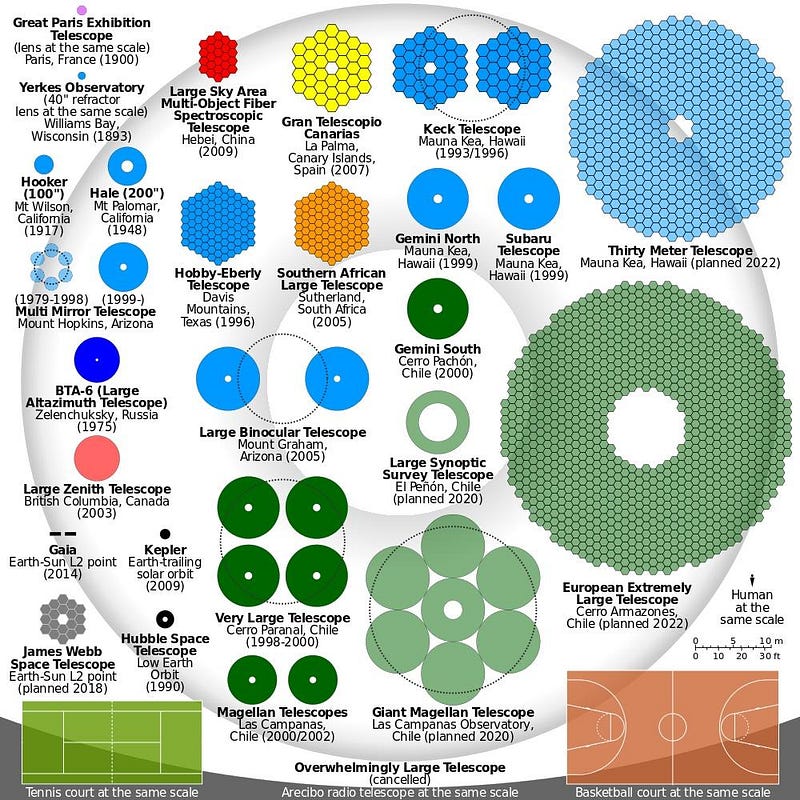
Transitioning from 10-meter to 30-meter class telescopes will yield approximately:
- 3 to 4 times higher resolution,
- 10 times greater light-gathering capacity,
- Enhanced adaptive optics compensating for atmospheric disturbances,
- Innovations in quantum optics enabling ultra-stable spectral recordings.
The European Extremely Large Telescope (ELT) is poised to make the first direct measurement of the Universe's expansion. With the recent discovery of numerous new ultra-distant quasars at varied redshifts—a trend anticipated to escalate with the Large Synoptic Survey Telescope—ELT's capabilities should allow for the direct detection of expansion.
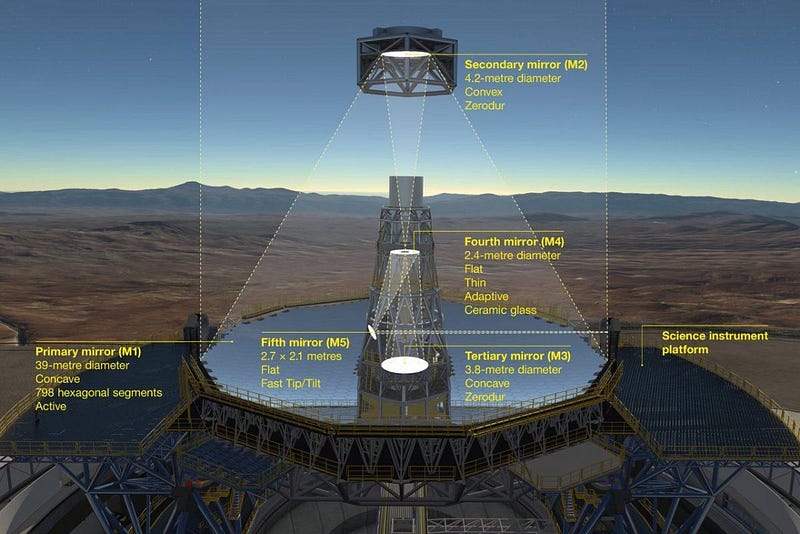
Expected to commence operations in the mid-2020s, the ELT should enhance redshift measurement precision by a factor of ten compared to existing instruments. With thousands of quasars anticipated to be accurately measured at the necessary distances, the ELT will be sensitive to minute changes in redshift corresponding to shifts of just 10 cm/s.
This advancement represents a tenfold to twentyfold improvement over current telescopes, meaning that within a decade or so after the ELT's full operation, we should have the capability to directly measure the Universe's expansion.
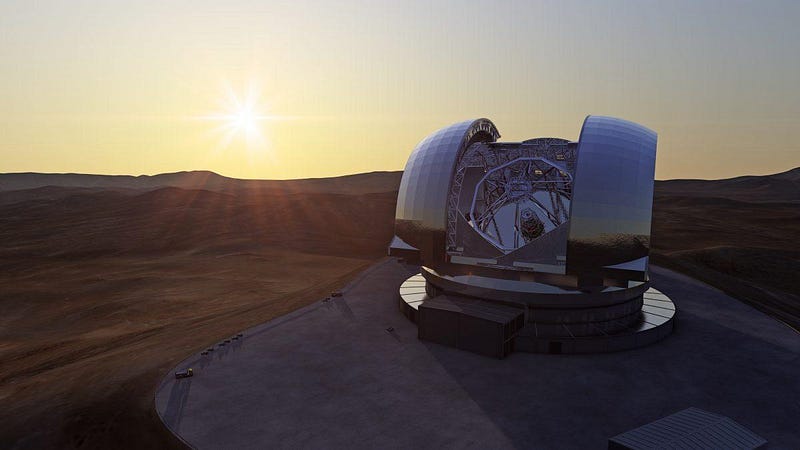
As we approach the mid-2030s, the term redshift drift will become crucial. By observing how cosmic redshifts evolve over time—a feat never before achieved—we will be able to explore numerous aspects of our Universe, including:
- Whether cosmic expansion aligns with theoretical cosmology predictions for a uniformly filled Universe governed by General Relativity.
- Whether dark energy acts as a true cosmological constant or varies in strength across time and distance.
- Whether changes favor a faster (73 km/s/Mpc) or slower (67 km/s/Mpc) expansion rate.
- Whether the flux from distant objects remains stable enough to detect any potential flux drift.
By 2040, we should be poised to directly confirm the Universe's expansion, rigorously testing our understanding of the cosmos.
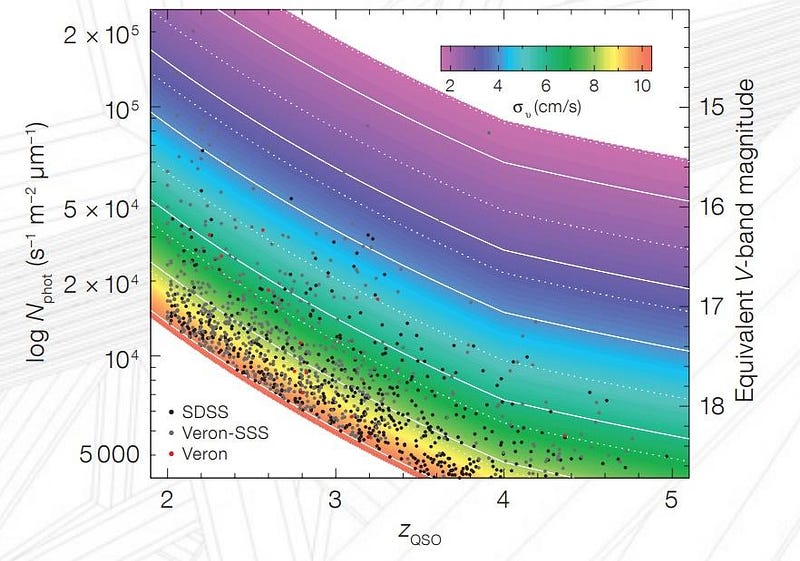
A common misconception persists regarding the risks of constructing larger, more powerful instruments to explore the Universe. The prevailing belief is that pursuing higher energies, lower temperatures, or larger apertures might yield fruitless results, squandering valuable resources.
In reality, pushing the boundaries of discovery is essential for acquiring new knowledge that drives technological advancements. While the outcomes of such explorations remain unpredictable, our focus should be on investing in the pursuit of the unknown and expanding our understanding of what is possible.
For nearly a century, we have recognized the Universe's expansion. In the coming two decades, we will gain direct evidence clarifying the mechanics behind this phenomenon.